News Story
UMD Bioengineers Take New Approach to Engineering Heart Tissue
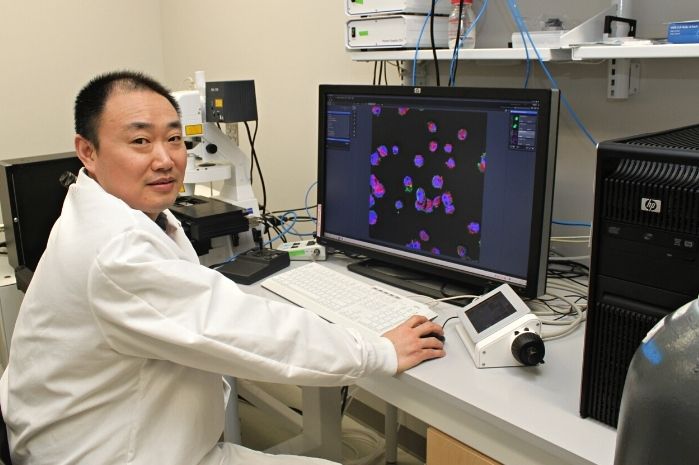
BIOE Professor Xiaoming (Shawn) He
University of Maryland professor Xiaoming (Shawn) He and fellow Fischell Department of Bioengineering (BIOE) researchers developed a new strategy to improve engineered heart tissues and one day advance overall understanding of heart disease.
According to the Centers for Disease Control and Prevention, heart disease remains the leading cause of death for both men and women in the United States. A major reason for this is that the human heart has very limited capacity to regenerate cardiomyocytes – the contractile cells of the cardiac muscle – when these cells become damaged. This damage commonly occurs due to ischemia – a reduction of blood flow to the heart, most often resulting from buildup or a blockage in the arteries.
Stem cell therapy is widely considered a promising strategy for treating heart disease. Given the significant ethical concerns associated with human embryonic stem cells, scientists look instead to use what are functionally embryonic stem cell-like cells – called human induced pluripotent stem cells (iPSCs) – to generate cardiomyocytes.
The science behind the process of tapping human iPSCs in this way is both fascinating and complex. For example, as an alternative to using human embryonic stem cells, bioengineers can take fibroblasts – the most common type of cell found in connective tissue – from a patient’s skin. They then “reprogram” these cells into iPSCs to convert – or differentiate – them into cardiomyocytes. To do this, historically, scientists have harnessed the power of viruses – such as adenoviruses – to transfer the genes they need into the harvested skin cells through the process of transduction. In this way, scientists can essentially “recode” skin cells to become cardiac muscle cells.
Unfortunately, the process poses many challenges. For one, the use of a virus or viral vector raises health concerns because of the potential for the virus to infect unintended cells in the human body. Even when scientists don’t use a virus in the reprogramming process, they face an additional hurdle. Believe it or not, iPSCs have the ability to transform into virtually any type of cell in the human body; this means that a human iPSC intended to be a cardiac muscle cell might become skin, hair, or bone instead.
Put simply: if the aim is to engineer cardiac muscle tissue, there is no room for error. A batch of human iPSCs intended for use in the heart simply cannot include an errant skin, hair, or bone cell.
But, the process of differentiating cells into cardiomyocytes can take time – as many as two weeks or more using traditional methods. In order to shorten the time frame and increase the yield of human cardiomyocytes, engineers often use 3D cell cultures rather than the conventional 2D approach. Even more, they use rho-kinase inhibitors – better known as ROCK inhibitors – to improve the survival of 3D-cultured human iPSCs before differentiating them into cardiomyocytes.
For years, engineers have accepted 10μM as the standard concentration of ROCK inhibitors for use in this process.
But years of hard work – and a little bit of luck – led Fischell Department of Bioengineering Professor Xiaoming (Shawn) He and a team of researchers to an important discovery. Their findings were published in Bioactive Materials.
He and his team found that the ROCK inhibitor commonly used in 3D cultures of human iPSCs contributed extensively to their heterogeneous differentiation. In other words, the ROCK inhibitor improved both the survival and yield of human iPSCs, but at a quality cost: the yields always included unintended cells, mixed in with cardiac muscle cells after cardiac differentiation.
“When put in 3D culture, human iPSCs easily undergo a type of cell death known as apoptosis, which can be minimized by adding 10μM ROCK inhibitor,” He said. “However, our work shows that this high concentration of ROCK inhibitor primes human iPSCs into not only the mesoderm for cardiac differentiation but also the other two germ layers –ectoderm and endoderm – for skin, hair, or bone differentiation, even before initiating cardiac differentiation.”
He and his team found that by reducing the concentration of the ROCK inhibitor to 1μM, they could dramatically improve the quality of human iPSC differentiation into cardiomyocytes. In fact, with this revised concentration, the team produced cardiac muscle cells with a beating efficiency of 95 percent in just a week, compared with when they used 10μM of the ROCK inhibitor; in the latter scenario, their yield of cardiomyocytes achieved a beating efficiency of under 50 percent in two weeks’ time. Even more, in the 1μM scenario, He’s team was able to synchronize the beating of their cardiomyocytes, creating a cardiac cell network – a cardiac spheroid – that beats at the same rate (~1 Hz) as that of the human heart. And, they did so with human iPSCs reprogrammed by a non-virus method.
“This paves the way toward producing a large number of highly pure and synchronized patient-specific cardiomyocytes for clinical use, with minimized concern on unintended tissue growth, arrhythmia, or viral infection,” He said.
Moving forward, He and his team hope to tackle the challenge of increasing their yield of 3D-cultured human iPSCs. Their work could help point to new techniques for large-scale production of human iPSCs-derived cardiomyocytes for use in engineering 3D cardiac constructs. Examples include bioprinting human hearts with therapeutic applications for heart attacks, heart transplantation, and high-fidelity drug screening of cardiotoxicity. The team’s work could also advance understanding of deadly heart diseases.
BIOE postdoctoral researcher Bin Jiang served as first author on the paper. In addition to Xiaoming (Shawn) He, BIOE postdoctoral researcher Wenquan Ou, Ph.D. students James Shamul and Samantha Stewart, visiting scholar Hao Chen, alumna Sarah Van Belleghem (Ph.D. '21) and BIOE chair John P. Fisher also contributed to the paper, along with Zhengou Liu, M.D., Ph.D. of the University of Missouri’s Division of Cardiovascular Medicine.
Along with this work, He's research group also develops novel strategies to bank human cells, tissues, and organs. Recently, they developed a novel natural sand-based technology to greatly improve the quality and yield of banking human iPSCs. This work is also published in Bioactive Materials.
In addition to his appointment as BIOE professor, He also holds affiliations with the UMD Marlene and Stewart Greenebaum Comprehensive Cancer Center and the UMD Robert E. Fischell Institute for Biomedical Devices.
Published February 11, 2022