News Story
The Future of Biohybrid Devices
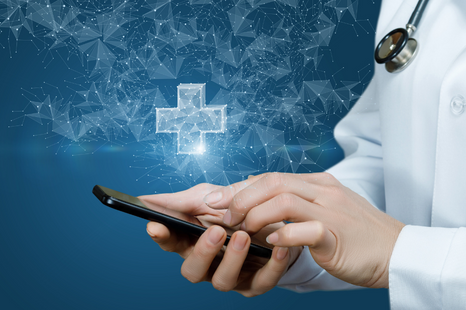
Imagine a world in which an app on your phone could pinpoint when you might expect to shake off that seasonal cold. What if that same app could monitor bacteria levels in your gut, where the microbiome helps regulate your immune system? Or, to take things a step further: what if the same app could actually trigger an immune response as soon as harmful bacteria are detected, sparing you from a bout of food-borne illness or the full brunt of a virus?
When it comes to medical devices, engineers have already developed technology that can transmit, receive, and process complex digital data into information everyday wearers can understand, nearly instantaneously. From the first pacemaker to today’s smart watches, medical devices and wearables have come a long way thanks to advances in this kind of technology. As for the human body itself? Each and every one of us benefits from countless cell-based “micro-processors” that prompt immune responses, send important signals to the brain, or accelerate wound healing. In both the electronic and biological worlds, decades of research and innovations enable scientists to understand a lot about how and why these systems work the way they do.
But, the biotech world hasn’t yet bridged the gap between the two realms.
That’s because modern electronic devices process information using materials such as silicon, gold, or chemicals, and an energy source that provides electrons; whereas, free electrons do not exist in biological systems. The human body simply cannot translate electronic signals into biological information that drives a biological response.
But, University of Maryland (UMD) bioengineers hope they might bridge that gap.
At UMD’s Robert E. Fischell Institute for Biomedical Devices, researchers have been working to develop a technique to translate biological information into electronic information, and vice versa. To do this, they’re hitching a ride with a small class of molecules known as “redox” molecules, which are capable of shuttling electrons to any location in the body. This process requires redox molecules to undergo a series of chemical reactions – oxidation or reduction actions – that enable them to transport electrons to the intended target in the body.
Recognizing this, Fischell Institute director William E. Bentley is leading a team that’s on a quest to tap redox-linked synthetic biology to enable this electron transfer to and from “smart” materials that microelectronic devices can read.
“We are combining the latest tools of synthetic biology, materials science, and multiscale modeling to develop innovative ways to sense, store, and retrieve biological information while also recognizing the wider context for that information,” Bentley said. “For example, if I have a low oxygen level, that’s one thing, but the same low oxygen level when I’m at the top of a high mountain is a different circumstance. The same oxygen level could be an indicator of poor health – at sea level – or nothing at all – on top of a mountain. Capturing context is what biology does well, but electronics does poorly. If we want to interconvert information, we need to capture and convey context.”
Bentley is collaborating with Fischell Institute Fellow Gregory Payne, as well as Georgia Tech professor of chemical and biomolecular engineering Corey Wilson and University of Wisconsin–Madison associate professor of medical microbiology and immunology Elebeoba "Chi-Chi" May. Together, they're working at the forefront of this new realm of redox-based bioelectronics. The group's long-term vision – backed by a newly awarded three-year, $1.5 million National Science Foundation (NSF) grant – centers on a future in which redox-based bioelectronics open entirely new lines of communication between the electronic and biological worlds. Their team goes by the name “TURIN,” an abbreviation for “Towards Understanding and controlling Redox for microbial memory and Interactions.” TURIN aims to advance the redox system the group has already developed with the help of an extensive network of collaborators, including leading minds in industry and federal agencies.
“We think there are huge opportunities for applications of this work that address our grand challenges in human health, sustainability, energy, security, and food,” Bentley said.
Bentley, Payne, and their team are supported by the NSF Semiconductor Synthetic Biology for Information Processing and Storage Technologies (SemiSynBio) program. SemiSynBio seeks to lay the groundwork for future information storage systems at the intersection of biology, physics, chemistry, computer science, materials science and engineering.
In addition to serving as director of the Fischell Institute, Bentley also serves as director of the Maryland Technology Enterprise Institute and as a professor with the UMD Fischell Department of Bioengineering. Both Bentley and Payne also hold appointments as UMD Institute for Bioscience & Biotechnology Research Fellows.
Published November 15, 2022